Let’s talk again about silver nanowires. First we discussed silver nanowires in batteries, now its time for sensors for human movement tracking. Not as some sci-fi McGuffin but as a real, bleeding-edge material reshaping how we track human movement. You want health monitoring, VR integration, gesture recognition, or next-gen prosthetics? You’re gonna want these microscopic silver threads in your life. And yeah, the tech actually works.
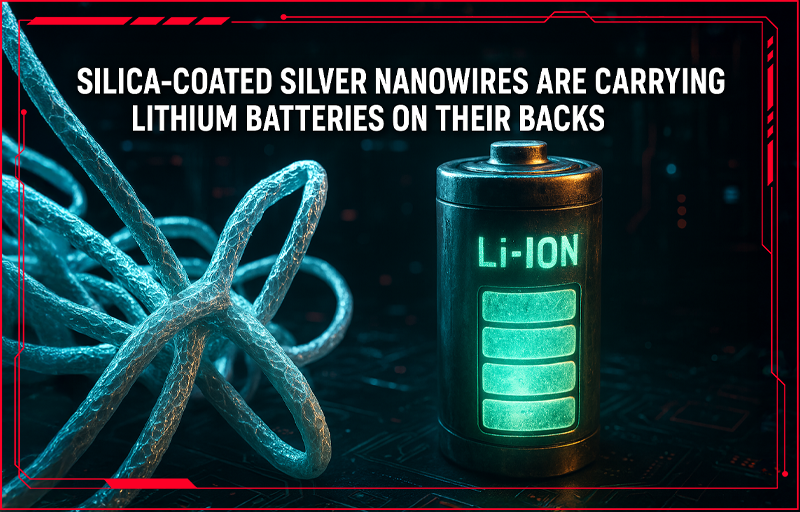
This isn’t white paper fluff! A 2024 paper from Mijit et al. lays out a full-on blueprint: a silver nanowire-based strain sensor system that’s not just flexible and sensitive, but also cheap enough to slap onto your elbow, neck, or even your forehead—and it’ll still supply precision waveform data like a champ.
What’s So Special About Silver Nanowires?
Let’s cut through the noise. Most “flex sensors” try to use exotic materials like graphene, MXenes, or carbon nanotubes. Problem? Those materials are fragile and fussy to manufacture at scale. Silver nanowires (AgNWs), on the other hand, check all the boxes:
- High electrical conductivity
- Wild stretch-ability (up to 660% strain in this study)
- Transparent and flexible
- Simple, scalable fabrication (drop-casting)
- Cheap materials compared to exotic nano-stuff
- Anti-Microbial properties
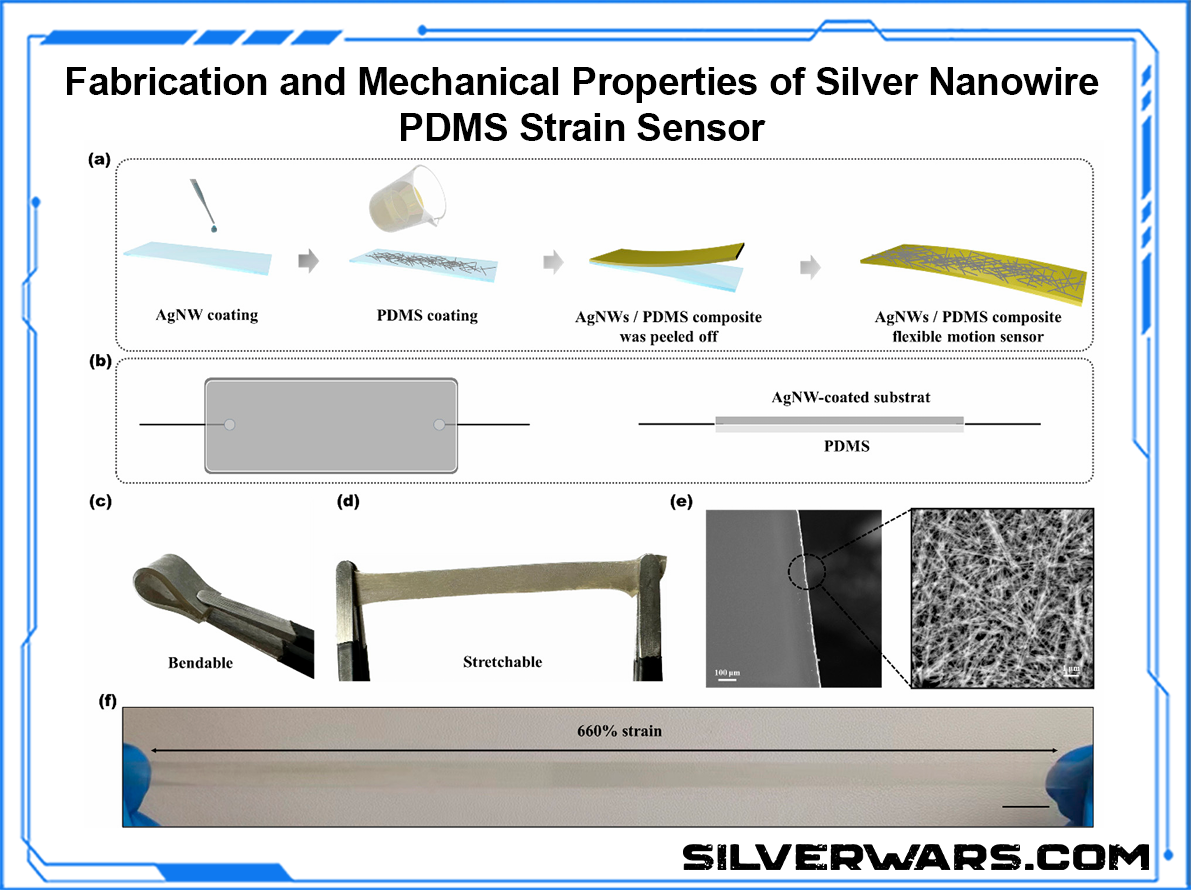
And unlike traditional sensors that either go brittle or start throwing junk data when things get moist or twisted, AgNW sensors are chilling through 5,000 deformation cycles without breaking a sweat.
Construction Without the Overhead
The fabrication process is laughably simple, especially compared to graphene-layer wizardry.
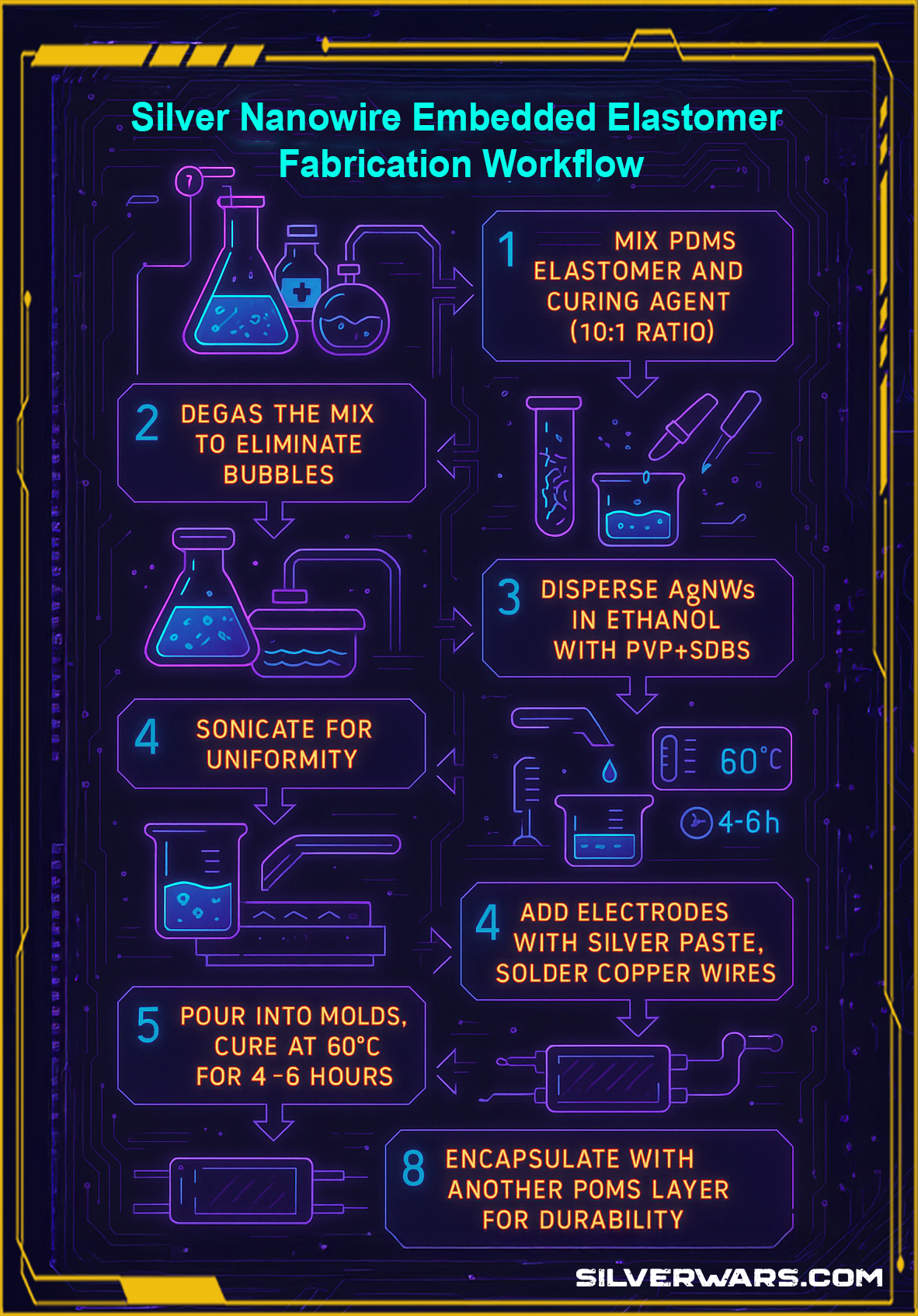
Step Processes
1 Mix PDMS elastomer and curing agent (10:1 ratio)
2 Degas the mix to eliminate bubbles
3 Disperse AgNWs in ethanol with PVP + SDBS
4 Sonicate for uniformity
5 Mix the AgNW solution into PDMS
6 Pour into molds, cure at 60°C for 4–6 hours
7 Add electrodes with silver paste, solder copper wires
8 Encapsulate with another PDMS layer for durability
No clean rooms. No semiconductor voodoo. Just chemistry, heat, and a little finesse.
Soft sensors detect movement by showing changes in electrical conductivity when stretched with conductive materials.
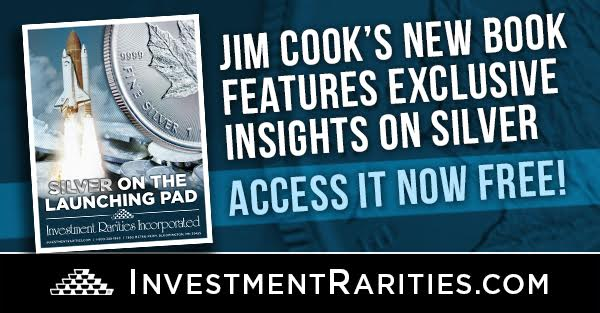
Performance Benchmarks That Actually Matter
Unlike some janky lab prototypes, this thing holds up under real-world pressures. It flexes like skin but reads strain like a data-sniffing bloodhound. The perfect invasive tool for our brave new world.
Gauge Factor & Responsiveness
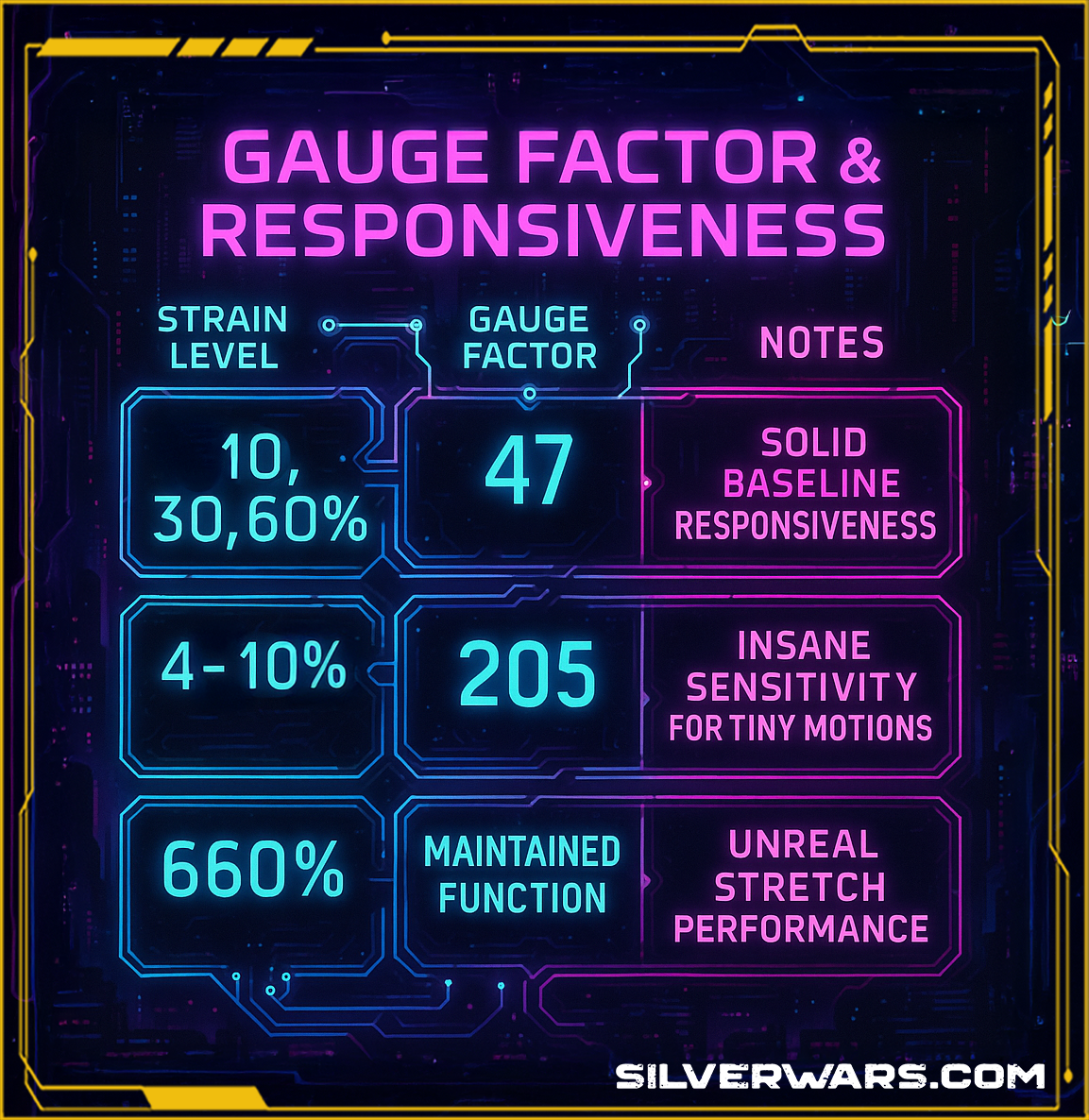
You don’t just get raw readings—you get consistent, linear, and repeatable response curves across motion frequencies (from chill 0.33 Hz gestures to 6.15 Hz rapid snaps). And it doesn’t fall off a cliff after extended use: 5,000 cycles in, and the sensor’s still jiving.
Material Comparison: PDMS vs PEA
They tested the silver nanowire sensors on different substrates to see which flexed better. PDMS—aka the GOAT—clearly outshines PEA.
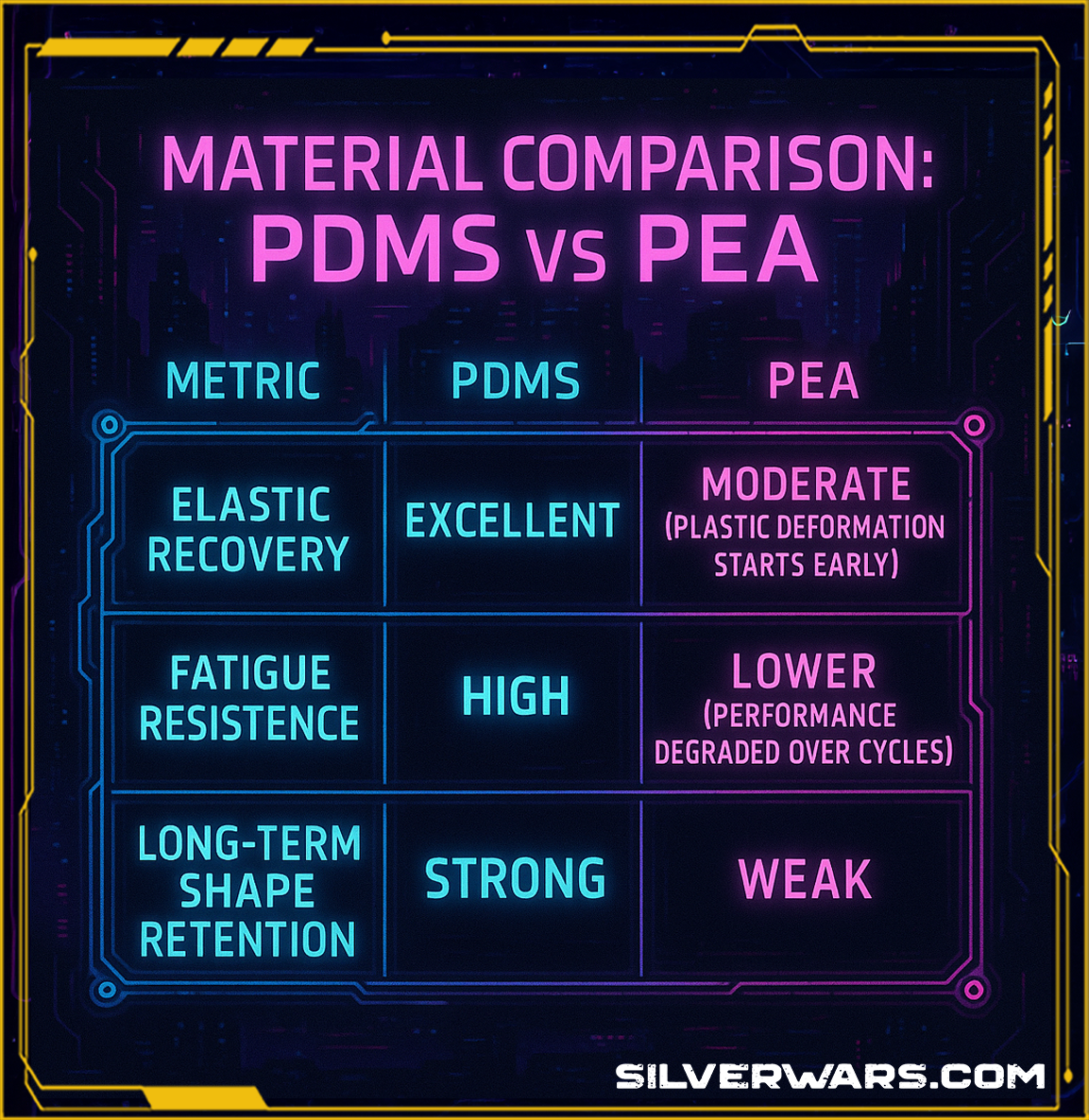
If you're designing a wearable or prosthetic application, it's PDMS or bust.
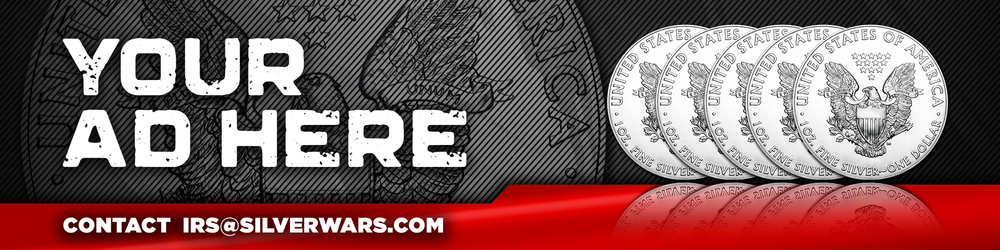
Density Matters (But Not Too Much)
This part’s smart: the researchers played with the density of silver nanowires in the matrix. Low-density sensors are better for general limb movement (like your elbow doing a bendy thing). High-density ones? They’re for micro-movements—like eyebrow twitches, finger sign language, or throat swallows.
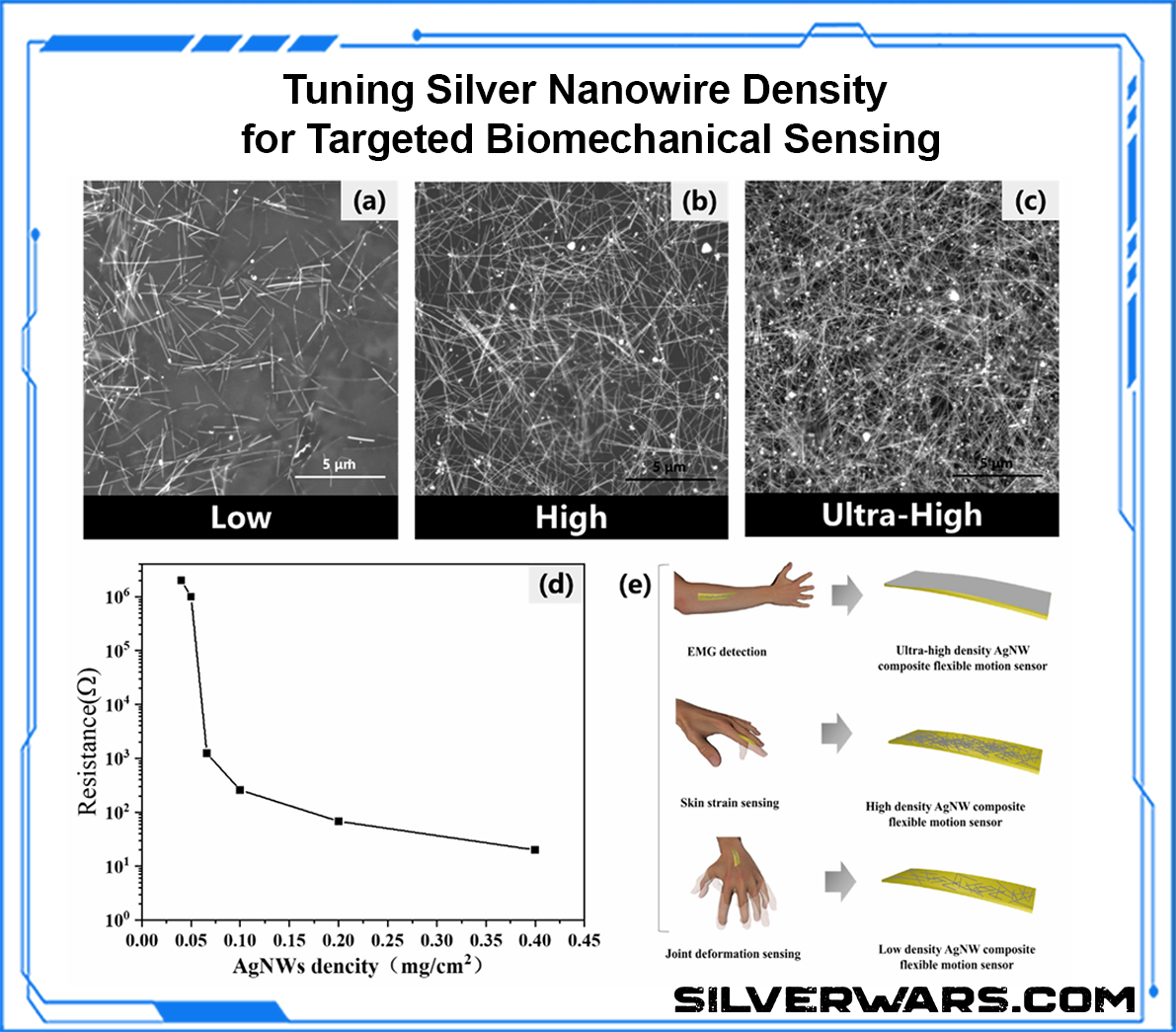
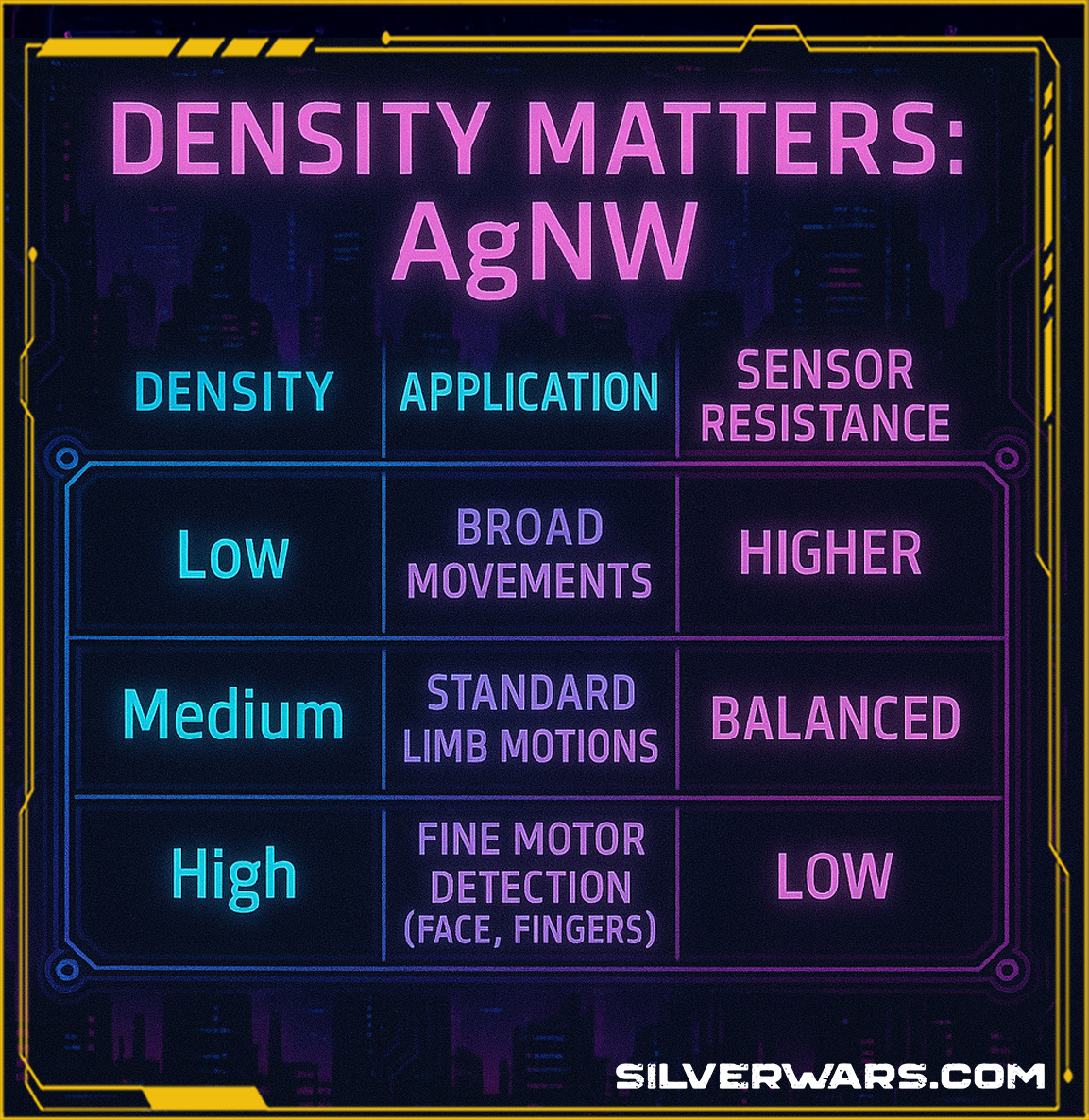
This modular density strategy means you could literally wire a whole-body motion capture suit—customized to each muscle group’s motion style.
Real World: From Elbows to “I Love You”
This wasn’t just a “lab results only” deal. They tested these sensors in vivo on actual human bodies.
Use Cases Demonstrated:
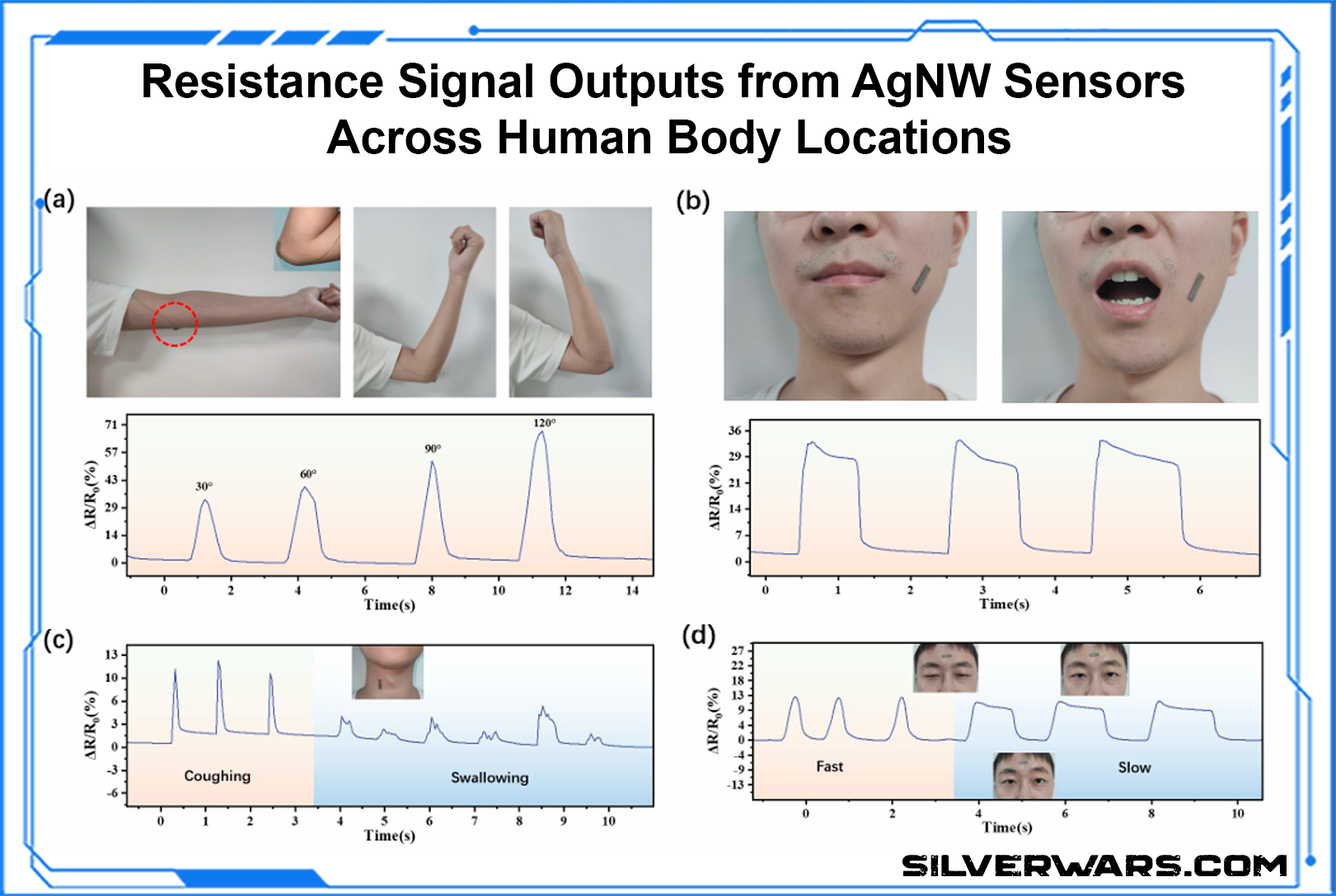
- Elbow flexing — Full waveform capture of arm bends
- Facial motion — Mouth movements and eyebrow twitches
- Throat tracking — Swallowing and coughing detection
- Finger gestures — Full recognition of sign language (they demo’d “I-L-U” for “I Love You”)
- VR gesture controls — Open palm = forward, clenched fist = brake, hand tilts = steering
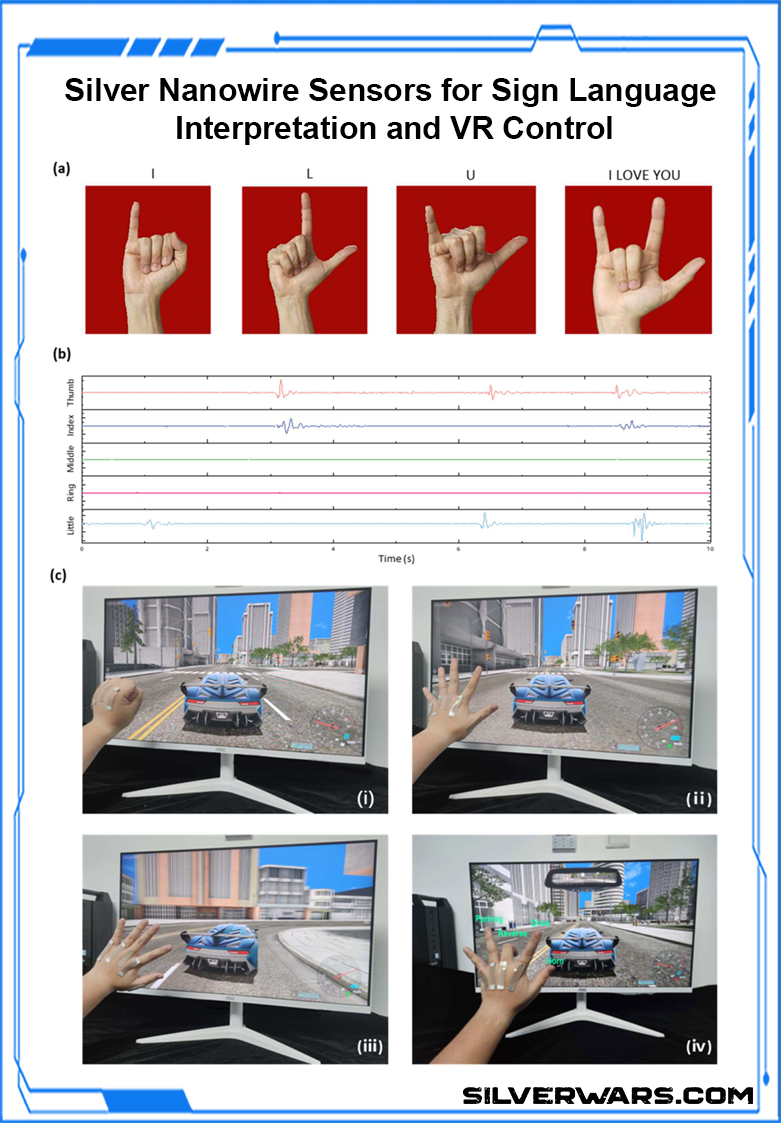
This opens the door for accessibility tools, VR interfaces, wearable biometrics, and more. Think gesture-controlled wheelchairs. Silent speech. Prosthetic control. Medical diagnostics based on cough patterns. The works.
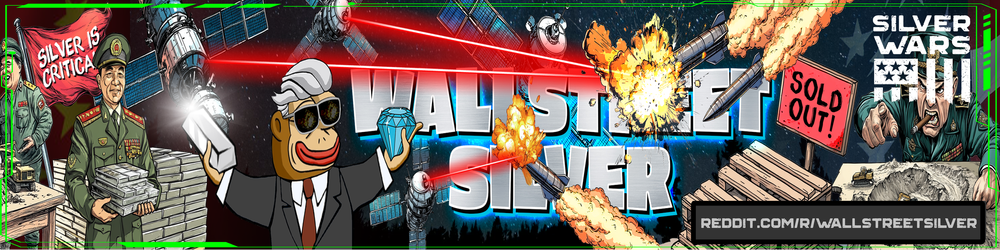
The Sensing Mechanism: Crackhead Physics (In a Good Way)
Silver nanowires don’t just sit there. They react.
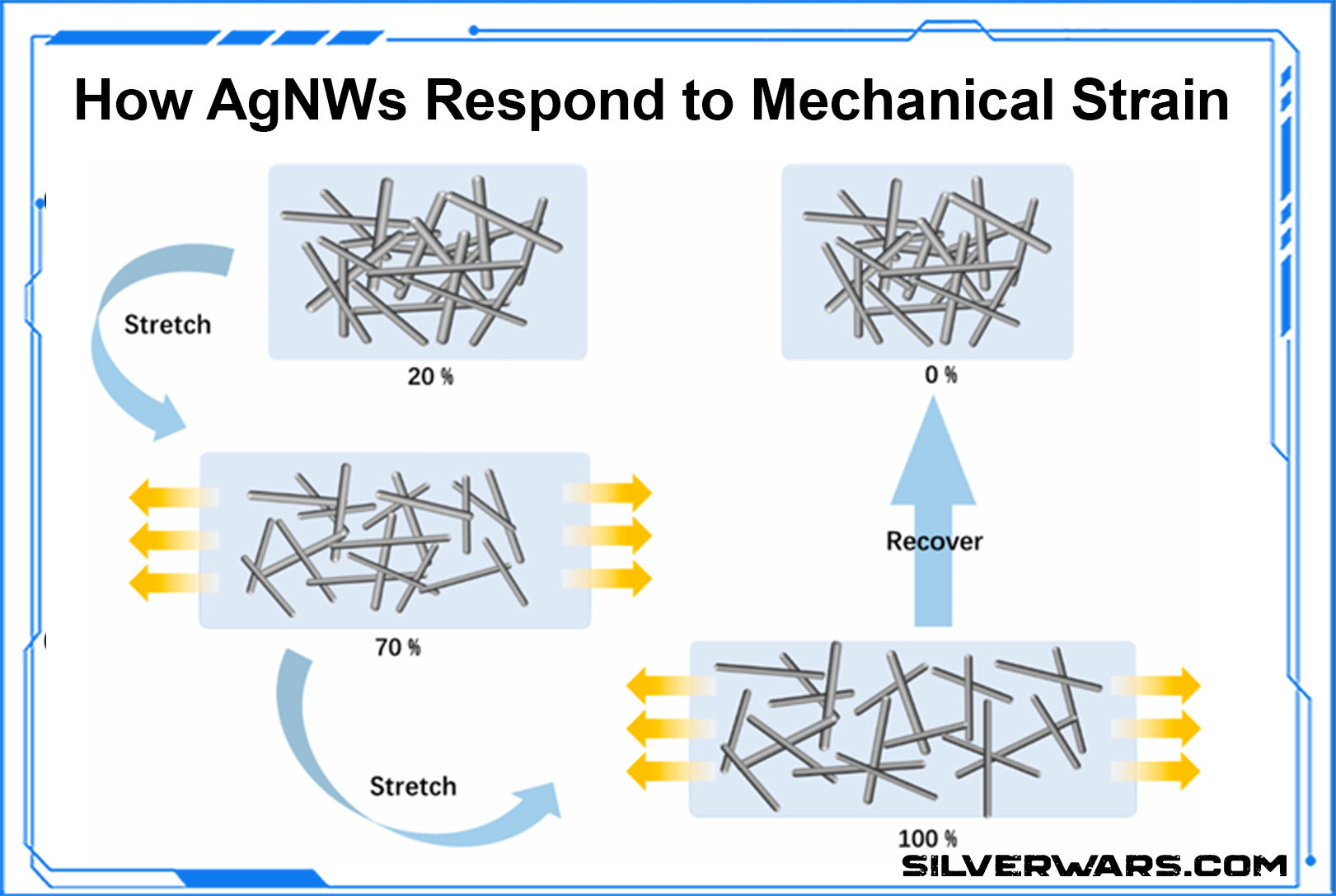
- 0–35% strain: Network starts thinning, tiny cracks form → resistance increases gradually
- 35–75% strain: Cracks multiply and widen → resistance spikes
- >75% strain: Full-on fracture zones, breaking up the network → huge resistance jump
That nonlinear resistance escalation isn’t a bug—it’s a feature. It allows the sensor to behave differently at different stages of strain, perfect for nuanced motion mapping.
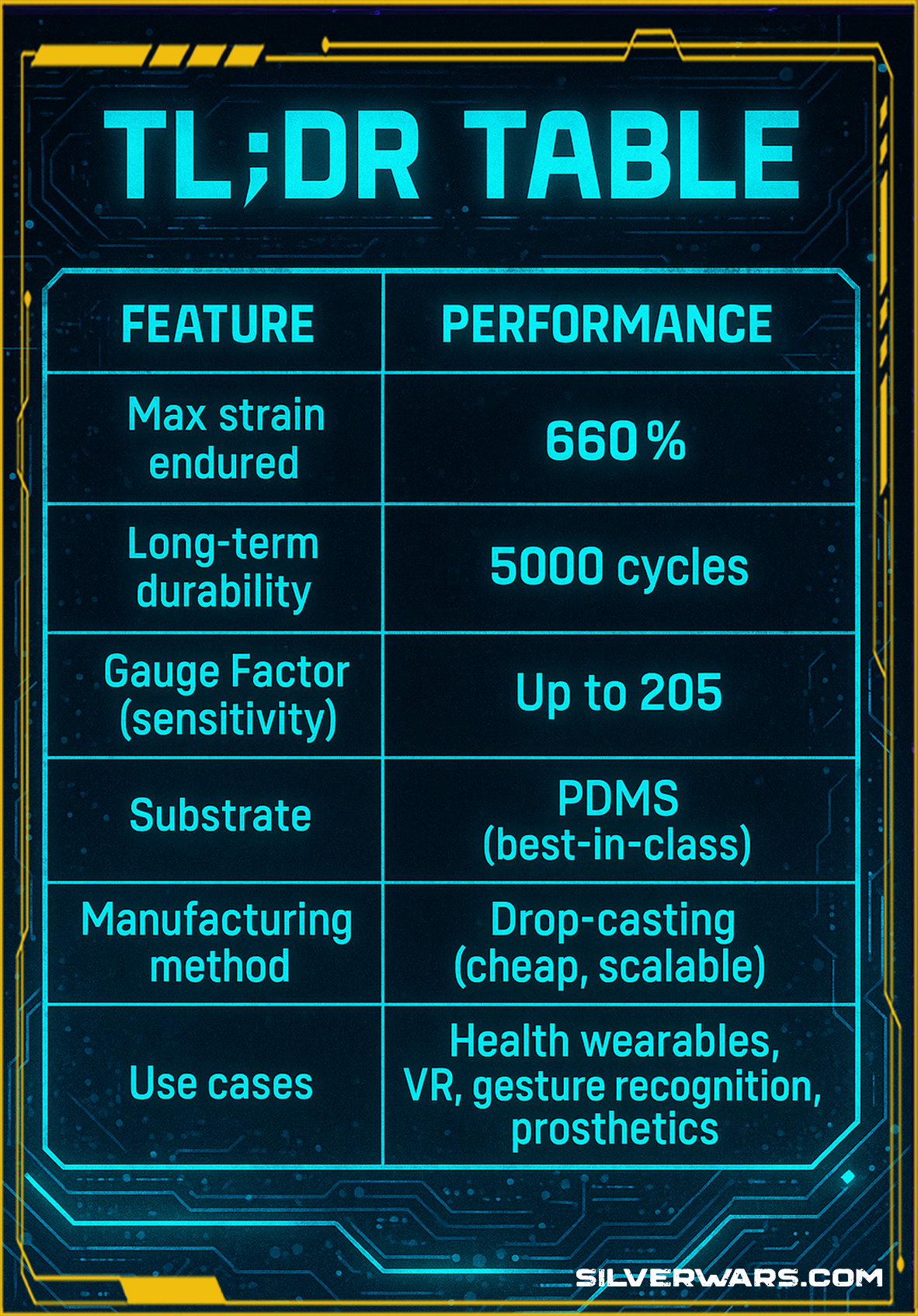
The Silver Wars Continue
This isn’t just a “cool tech” footnote. This is an actual leap forward in how we mesh hardware with the human body. These silver nanowire sensors are cheap, tough, hyper-sensitive, and easy to produce.
The silver isn’t just shiny—it’s useful. It’s redefining wearables from “clunky step counter” to “real-time biomechanical readout.” And the best part? It works without needing a PhD to build or interpret.